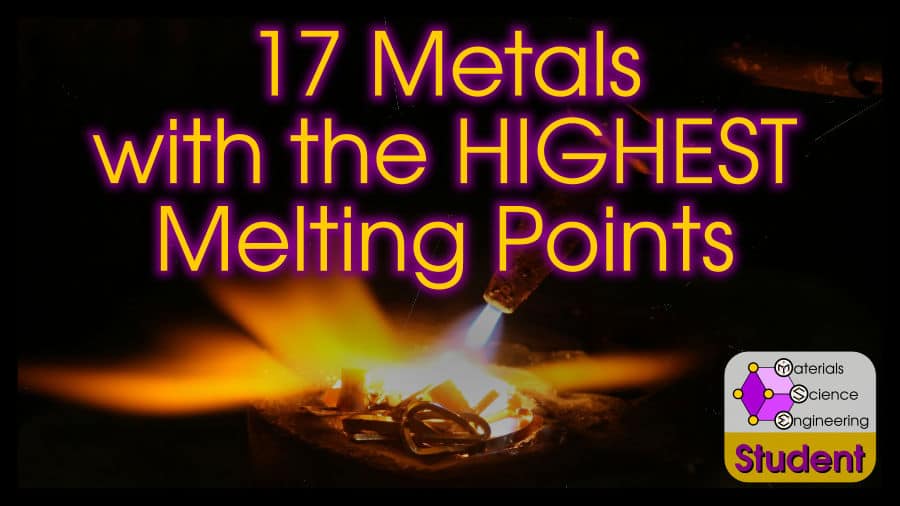
Did you know that tungsten melts more than 3400°C (6200°F) higher than mercury? Tungsten is the metal with the highest melting point, but why does it take so much energy to transform tungsten from solid to liquid?
The melting point of a material is primarily related to bond strength. Materials with strong bonds between atoms will have a high melting temperature. However, other factors–such as crystal structure, atomic weight, and electron structure–can also influence the melting point.
But before we dive into an article focused on metals, I want to quickly point out that metals don’t necessarily have the highest melting point when compared to other materials.
Metallic bonding is definitely stronger than Van der Waals bonding, but ceramics (which have ionic or covalent bonding) have even higher melting points! Graphite, hafnium carbide, tantalum carbide, and other ceramics have even higher melting points than tungsten!
Outline
List of High Melting Point Metals
Before I get into a scientific explanation, here’s that list of those 17 elemental metals with the highest melting points. (I’ve also listed the crystal structure, which will come up later). As you can see, tungsten is #1 with an astonishing 3380°C melting point.
- Tungsten (W)
3380°C, BCC - Rhenium (Re)
3180°C, HCP - Osmium (Os)
3027°C, HCP - Tantalum (Ta)
3014°C, BCC - Molybdenum (Mo)
2617°C, BCC - Niobium (Nb)
2468°C, BCC - Iridium (Ir)
2447°C, FCC - Ruthenium (Ru)
2250°C, HCP - Hafnium (Hf)
2227°C, HCP - Technetium (Tc)
2200°C, HCP, Radioactive - Rhodium (Rh)
1963°C, FCC - Vanadium (V)
1902°C, BCC - Chromium (Cr)
1857°C, BCC - Zirconium (Zr)
1852°C, HCP - Platinum (Pt)
1769°C, FCC - Thorium (Th)
1755°C, FCC, Radioactive - Titanium (Ti)
1670°C, HCP
High Melting Point Alloys
High melting point alloys are actually not a real category of material. Any time you combine metals in solid solution (i.e. make an alloy), the melting point will decrease.
If you wanted a metal with a high melting point, you’d probably have to choose a pure metal. In many cases you could probably get away with slight alloying from one of the pure elements listed above, but it’s not really possible to engineer alloys for increased melting point.
“High temperature” alloys are a real category in materials science. In most cases, materials fail in high temperatures waaaay before they would melt. High temperatures make metals softer and more susceptible to oxidation or corrosion.
This article is purely about the phenomenon of metals that have high melting points, but superalloys (Ni, Co, or Fe based, 1300-1500°C in the upper range), titanium alloys, and tungsten alloys can be considered high temperature alloys because of their combination of high melting point, good strength at high temperatures, and oxidation/corrosion resistance
What Is the Reason for High Melting Points?
The simple answer is: bond energy.
A solid can be visualized as a group of atoms bonded together that vibrate back and forth, but generally stay in the same position.
The vibration of the atoms–their kinetic energy–is what we normally call “temperature.” Higher temperature means that the atoms vibrate faster. At some point, they vibrate so quickly that they can break their bonds and slide past each other.
Each atom has a different vibration, so even in the solid state there are some atoms which are able to temporarily break their bonds and move through the material. This phenomenon is called “diffusion.”
“Melting” is when most of the bonds break. In crystalline solids like metals, all the bonds are the same length–and strength–so there is a precise point where almost all the atoms gain enough thermal energy to break their bonds. The more energy it takes to reach that point, the higher the melting point.
So when do the atoms reach this point?
The most obvious factor is the direct bond strength. Bond strength is a bit hard to quantify, but two measures of bond strength are the enthalpy of formation, which is the enthalpy change to form a mole of material, and bond dissociation energy, which is the energy released from breaking a bond between two atoms.
In Callister’s table of bond energies, the textbook is actually tabulating enthalpy of formation. Here is a graph of bond strength (represented by enthalpy of formation AND bond dissociation energy) compared to melting point.
Now, there are a few more properties that influence the melting point, but wouldn’t be considered in the enthalpy of formation or bond dissociation energy. For example: how many bonds surround the atom?
In general, we consider that most effects from bonds are the result of an atom’s nearest neighbors, or the ones directly “touching” it. (Although there is an effect from atoms nearby as well–for example, if you make nanoparticles with only a few atoms altogether, the bonds are fewer than usual and the nanoparticle will have a lower melting point than the bulk material).
An element’s crystal structure determines the bond length and number of nearest neighbors (also called “coordination number”). This influences the bond strength in a way that is not captured by bond dissociation energy, but is reflected in melting point.
That’s why elements with high melting points tend to have crystal structures with high packing: FCC, HCP, or BCC. In fact, the elements with the highest melting point usually have a BCC structure. BCC is almost close-packed so it’s quite stable, but I suppose the slightly lower density allows the atoms to wiggle more without needing to liquefy. For a (very complex) mathematical proof of why BCC tends to be the most stable high-temperature phase, check out this paper by Alexander and McTague.
Because metallic bonding means atoms are surrounded by a sea of electrons, there are also a few characteristics of the electronic structure of the atom which can also impact bond strength as measured by melting.
For example, you’ll notice that many of the metals with high melting points have partially full d-suborbitals.
That means these atoms have a lot of electrons to contribute to the sea of electrons, generally increasing the interactive forces in the metal.
Larger atoms also tend to be packed more tightly and weigh more. Since kinetic energy is , heavier atoms will be vibrating more slowly at the same temperature as a lighter atom. Slower vibrations means that atoms will have more time to interact with each other, which may also contribute to a higher melting point.
What Are Common Properties of High Melting Point Metals?
Since I’ve chosen 17 elements with the highest melting point, these elements are quite diverse. Their melting temperatures range from 1670°C and 3380°C, so they don’t have that much else in common.
However, they all have BCC, HCP, or FCC crystal structures, which are the closest-packed, most stable crystal structures.
The metals from my list with the highest melting point are also refractory metals. These are a special kind of metal with a specific set of properties. You can read all about refractory metals in this article, but in short, they are:
- Dense
- Hard (especially tungsten and rhenium)
- Chemically inert
- Easily oxidized
- Creep resistant
The refractory metals also have a BCC crystal structure (except for rhenium, which is HCP).
Applications of High Melting Point Metals
There are many applications that require metals with high melting points. For applications like incandescent light bulb filaments, which just require a high melting point metal and nothing else, tungsten is usually the metal of choice.
Tungsten is the metal with the highest melting point. It is also relatively cheap, so there isn’t really an argument to use “cheaper” metal with a slightly lower melting point. Elements like rhenium, tantalum, and molybdenum are typically used because they have some other properties in addition to high melting point (for example: lower density).
Tungsten (W)
The metal that can withstand the highest temperature is not especially expensive either, so tungsten is the go-to metal for high-temperatures applications such as light bulb filaments, welding electrodes, and furnace heating elements.
Rhenium (Re)
Used in superalloys for jet engines to slow down diffusion (basically 70% of extracted rhenium is used for this purpose). It’s a rare/expensive element, so it’s rarely used as the main base metal in an alloy.
Osmium (Os)
Actually, its applications have almost nothing to do with its high melting point because osmium oxide is super toxic. However, it can be extremely hard/wear resistant at regular temperatures–for example, you may have used an alloy of osmium and iridium for fountain pen tips.
Tantalum (Ta)
Tantalum is used to make high temperature, corrosion resistant alloys, such as ones used in vacuum furnace parts.
Molybdenum (Mo)
Like many other materials on his list, molybdenum is primarily used to alloy with other metals. It sees significant use in superalloys for jet engines. It is a useful alloying element because it is relatively lightweight. Mo and Nb have the best combination of low density and high melting point.
Niobium (Nb)
Niobium is an element used in superalloys. Nb-based alloys are also used in aerospace engineering as materials for thruster nozzles in liquid rockets.
Iridium (Ir)
There are spark plugs based on iridium that are used in aviation. It is mostly used in electronics-related applications.
Ruthenium (Ru)
When it comes to high-temperature applications, ruthenium is used as an alloying addition to superalloys. Ruthenium may also be alloyed with platinum to increase platinum’s wear resistance, without losing platinum’s other properties.
Hafnium (Hf)
Like many other materials on this list, hafnium is primarily used to alloy with other metals like Fe, Ti, Nb, Ta, and others. C103 alloy, consisting of Nb (89%), Hf (10%) and Ti (1%), was used in liquid rocket thruster nozzles of Apollo Lunar Modules main engine. Sometimes it is added to nickel-based superalloys to enhance adherence of protective oxides layers.
Technetium (Tc)
Technetium is radioactive, which kinda limits its applications. However, it can be used for medical radioactive imaging, and it can also be alloyed with steel to improve corrosion resistance. You just need to be sure this steel is in a place where humans can’t get to!
Rhodium (Rh)
Rhodium is a noble metal that rarely reacts with other chemicals, which can make it a good catalyst. Like Pt, Rh is used for wires that may be exposed to a hot/corrosive environment. Pt can survive high temperatures and corrosion without alloying, which lets it preserve its electrical conductivity. Rhodium is even rarer than platinum.
Vanadium (V)
Vanadium is used in jet engines as an alloying addition to engineering titanium alloy (Ti-6Al-4V). One vanadium-based alloy (V-4Cr-4Ti) is a promising candidate to be used in future fusion reactors
Chromium (Cr)
While chromium has a high melting temperature, it is more known for its low density and oxidation resistance. Cr is the main alloying element (besides iron) in stainless steel, and it is alloyed in superalloy turbines. Even if high temperature alloys don’t directly contain Cr, they may have a thin coating of Cr-alloy for oxidation resistance.
Zirconium (Zr)
When it comes to high temperature applications, zirconium is widely used in the form of zirconium oxide. YSZ – ZrO2-8 wt% Y2O3 (so-called Yttria-Stabilized Zirconia) is a commonly used protective coating which aim is to increase maximum operating temperature of superalloys used in jet engines.
Platinum (Pt)
Platinum is a noble metal that rarely reacts with other chemicals, which can make it a good catalyst. Among high-temperature applications, Pt is used for wires that may be exposed to a hot/corrosive environment. Pt can survive high temperatures and corrosion without alloying, which lets it preserve its electrical conductivity.
Thorium (Th)
Thorium is radioactive. Nevertheless, thoria (thorium dioxide) has the highest melting point of all known oxides – 3300°C. Thus, it can remain solid in a flame and be used in gas mantles.
Titanium (Ti)
Titanium is used in compressors of jet engines (Ti-6Al-4V).
Final Thoughts
There you have it! The 17 metals with the highest melting point–generally speaking, because they have the strongest bonds.
A specific subset of this group with the highest melting points, such as tungsten, rhenium, and tantalum, are called refractory metals. You can read all about refractory metals by clicking this article!
References and Further Reading
For a (very complex) mathematical proof of why BCC tends to be the most stable high-temperature phase, check out this paper by Alexander and McTague.
In this paper, we found information on the correlation between bond energy and melting temperature for different elements.
Bond dissociation energies for different elements were taken from this paper.