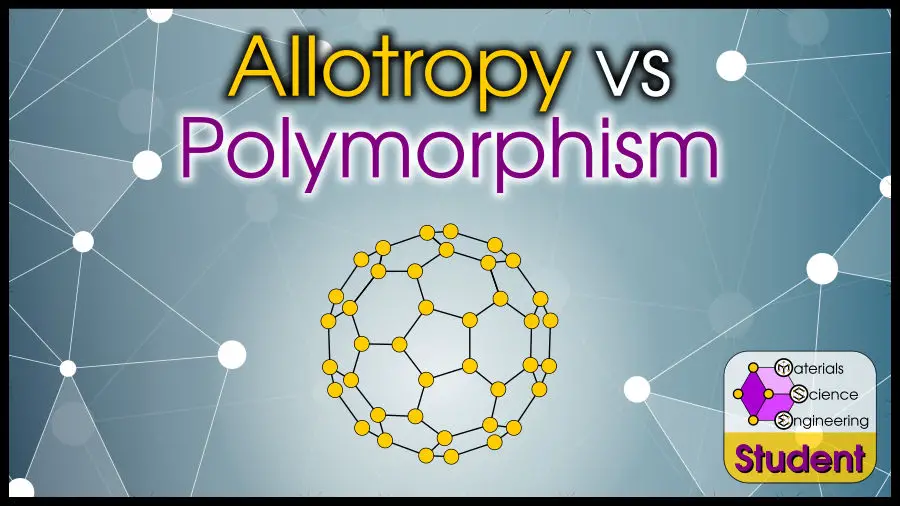
The concept of “polymorphism” is the closest that most high school chemistry classes ever come to materials science. And, like most simple concepts in materials science, polymorphism–and its more specific form, allotropy–are often misunderstood.
Polymorphism means that a material can exist in more than one solid-state (crystal) structure. Allotropy is a subset of polymorphism that only applies to pure elements.
Outline
What is Allotropy?
Allotropy is just polymorphism but for a pure element. It’s a great vocabulary word for know-it-alls to throw out when someone mentions polymorphism for an element.
“But actually,” you can say with the smug smile of someone who knows they are about to kill the mood, “it would be more accurate to say ‘allotrope’ because you’re talking about an element and ‘allotrope’ is the more specific version of ‘polymorph’ that only applies to pure elements.
You should try it once, so you feel that you put the knowledge on this page to good use, but only once.
Anytime you see the word “polymorph,” everything I write also applies to “allotropy” except that polymorph is more general. With that said, let’s get into polymorphism.
What is Polymorphism?
If you know your Greek, you can figure out the meaning of “polymorph” just by looking at it. “Poly-” means many and “morph” means form. So polymorphism is the ability of a material to take multiple forms (it can even be used in computer science, but about code instead of matter).
Of course, we now need to specify what counts as a “form.” Most materials can exist in solid, liquid, and gas states, so we need to define form more strictly. In this case, we mean that a material can exist as multiple kinds of solids.
Yes, actually, there are many kinds of solids. Unlike liquids and gases, in which atoms are arranged randomly and constantly moving around, most solids exist as crystals. That means the atoms are arranged in a particular repeating order, and they mostly stay in the same place.
Here, let’s take a look at the real phase diagram of water.
You see all those roman numerals in the blue area? Those are each a different crystal arrangement of ice (polymorph, not an allotrope, because H2O is not a single element). In chemistry class you probably just labelled the whole region “solid” because chemists are afraid of solids!
There are hundreds of crystal structures that your chemistry class never talked about. But before I talk about those, let’s use an example you probably have heard about: carbon.
Polymorphs of Carbon
“Hold up!” I hear an imaginary person asking. “Isn’t carbon an allotrope?”
Well, actually carbon is just an element. But arrangements of carbon atoms, like diamond, graphite, and buckminsterfullerene, are allotropes. But since all allotropes are polymorphs, these carbon crystals are also polymorphs.
Yep, carbon is a crystal, too! At least, graphite and diamond are crystals. Buckminsterfullerene, or buckyballs, are molecules. But molecular solids still count as a polymorphic solid form.
Graphene, incidentally, is not a polymorphic form. Graphene is just a single layer of graphite, so it still has the exact same atomic arrangement.
But between the other solid forms of carbon: graphite, diamond, and buckballs, the different atomic arrangements lead to very different properties.
Buckyballs can be expanded into nanotubes, diamond is the strongest naturally-occurring material, and graphite is relatively soft.
Non-Carbon Polymorphism
Okay, so I know you’ve heard about carbon allotropes before. You’re searching the web for stuff you didn’t already know, and I’m here to deliver.
There are many kinds of crystals, but three of the most common ones are body-centered cubic (BCC), face-centered cubic (FCC), and hexagonal close-packed (HCP). These are common because they are some of the densest ways to arrange atoms.
Each of these different crystal structures will give the atoms different properties. For example, FCC is denser than BCC. BCC crystals also have a ductile-to-brittle transformation temperature (DBTT), which means that if you built, say a ship (*cough cough* Titanic) out of a BCC steel instead of an FCC steel, and the ship ran into something cold, it would behave like a ceramic instead of a metal.
Iron can be allotropic. Just look at the phase diagram below! (Phases are states of matter + solid polymorphs) This graph tells you the most stable allotrope of iron at a particular temperature and pressure. Each of those greek symbols is a different crystal structure.
Let’s focus on reasonable pressures and temperatures (let’s say atmospheric pressure and up to 1500°C). The critical allotropes are ⍺ and γ. ⍺ is BCC and γ is FCC.
Suppose you took a wire of iron and heated it up. At first, the length of the wire would expand due to thermal expansion. But at about 1390°C, when the BCC iron turns into FCC iron, the wire would dramatically shrink because FCC is a more dense way to pack atoms than BCC.
Why Does Polymorphism Matter?
You see what I did there? “Matter?” Like, the stuff which can be a polymorph? Okay I’ll stop.
I gave you a seemingly inconsequential example with iron just a moment ago with iron, but these allotropes of iron can make a big difference to scientists.
But what about regular people?
Did you know that if you put a diamond in the oven, it will turn into graphite? That’s polymorphism (or allotropy) at work.
Thermodynamically, graphite could arrange itself in a diamond structure or a graphite structure. As it turns out, graphite is the more stable form. If you give diamond enough thermal energy for the atoms to make their own decisions, they’ll choose to form graphite.
Did you know that Napoleon failed his invasion of Russia because of polymorphism? (According to one of my old professors.)
When Napoleon invaded Russia, the soldiers’ tin buttons disintegrated in the cold. They had to survive the Russian winter with open jackets!
It turns out that the jackets on Napoleon’s army had tin buttons. At regular temperatures and pressures, tin is a ductile metal with a body-centered tetragonal structure. At cold temperatures, however, tin turns into the same crystal structure as diamond.
Unfortunately, diamond cubic is a much less-dense way to pack atoms, so there is a huge volume expansion when tin cools down. Tin can’t survive the stress from this large volume change so it basically disintegrates.
When Napoleon invaded Russia, the soldiers’ tin buttons disintegrated in the cold. They had to survive the Russian winter with open jackets!
Polymorphism, and phase transformations in general, are used in almost all engineered materials.
Can Glass be a Polymorph?
Oh, boy. Someone wants to show off their materials science knowledge.
All materials are either a crystal or a glass. The opposite of a crystal solid is an amorphous solid (that means glass). In a glass, the atoms are not arranged in a particular order. They organize themselves randomly, like a liquid stopped in time.
Glass occurs due to kinetics, not thermodynamics. Glass occurs because the atoms freeze before they can crystallize–they lose the energy to move around and essentially get trapped in a disorganized structure.
As far as I am aware, there are no thermodynamically stable amorphous solids (not counting polymers Mr. Know-It-Al!). If you took a liquid and cooled it infinitely slowly, it would always form a crystalline arrangement.
The question is, how fast do you need to cool it in real life? Some materials, like SiO2 (regular window glass), need to be cooled very slowly to form a crystal structure. But it can happen–crystal SiO2 is just quartz! On the other hand, most metals need to be cooled in picoseconds to “outrun” the atoms’ tendency to arrange in a crystal.
Since glass is not a thermodynamically stable phase, it is not considered a polymorph or allotrope. However, if you did find a material for which an amorphous state had the lowest thermodynamic free energy, then that amorphous state would be a true polymorph.
Final Thoughts
All this is to say the polymorphism and allotropy are basically the same thing. Allotropy is just a more specific version of polymorphism, where the material is only made of a single atom.
Either way, both allotropes and polymorphs are alternate ways that atoms can be arranged in the solid state. Polymorphism is extremely common in materials science and extremely important when considering engineering processes.
References and Further Reading
If you want to know more about the crystal structure differences in steel, this article explains all the differences between FCC vs BCC crystal structures!
If you want to know the differences between allotropes and isotopes, check out this post!