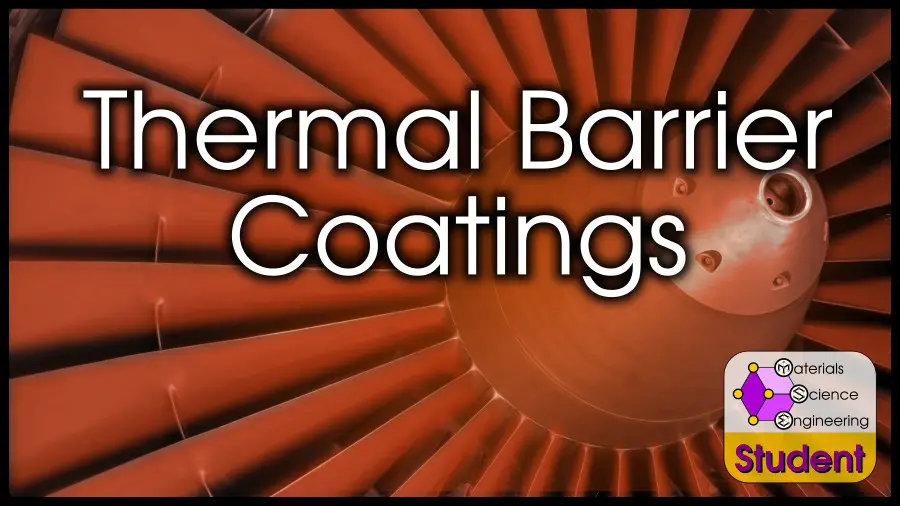
Thermal Barrier Coatings (TBCs) are heat-resistant, ceramic coatings that are applied to superalloys. TBCs have complex chemistry that protects the base superalloy from heat, oxidation, and corrosion. This can increase the operating temperature of the base superalloy by several hundred degrees.
Nickel-based superalloys with thermal barrier coatings are currently the most advanced materials for high-temperature applications. In this article, we’ll begin by explaining why high-temperature materials are useful, and then explain the role that TBCs play. We’ll also explain how TBCs work, how to make/process them, and some common materials for TBCs.
Outline
Why are High-Temperature Materials Important?
My PhD research is related to high-temperature materials, so obviously I think this is really important. However, I realize that many of my readers just want some information on TBCs so they can complete a school project, so I’ll hide this information behind collapsable text.
Click here to find out more.
High-temperature materials are important because they represent a way to break one of humanity’s limits. If we want to run an experiment, measure geological processes, or explore space, we may be limited by temperature.
In fact, temperature is a more limiting factor than most people realize. Have you heard of the SR-71, the famous “blackbird” spy plane that can travel at more than 3 times the speed of sound? That plane heats up several hundred degrees, just because of wind resistance. The plane is not even airtight at room temperature! There are gaps in the design to accommodate thermal expansion, so the plane actually needs to be heated before it can take off!
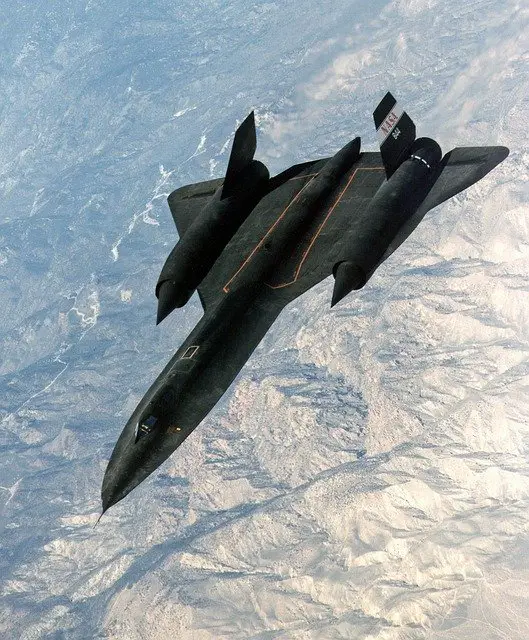
The most commercially important reason to have high-temperature materials is for energy conversion. Many power plants use gas turbines, which operate on the principle of Carnot efficiency. Basically, that means that–the hotter you can run the turbine–the less energy you waste when converting fossil fuels to electricity.
As you can see from the graph above, an increase in temperature provides a bigger increase in power, because of the efficiency limit. With world energy consumption on the order of 1020 joules per year, even a 1% increase of efficiency is a multi-million dollar problem. Ultimately, these turbines are limited by materials science. We would love to run hotter engines, but the metals can’t survive those temperatures.
The current solution is nickel-based superalloys. These alloys do not have the highest melting point, but they have a unique microstructure that allows them to stay strong at a high fraction of their melting point.
Thermal barrier coatings are the next step beyond superalloys.
Why Do We Need Thermal Barrier Coatings?
Superalloys have many fantastic properties–there’s a reason we call them “super!” While the bulk of a turbine blade is made of superalloys, TBCs are a coating applied to the surface. This coating can provide several improvements to the underlying superalloy.
TBCs are a ceramic, which means they tend to be harder and stronger than metals (but more brittle, which is why you can’t make the whole blade out of a ceramic!). Ceramics usually have better oxidation- and corrosion-resistance than metals. They also have much higher melting points.
By placing a ceramic coating on a superalloy, you can combine the best properties of both.
By placing a ceramic coating on a superalloy, you can combine the best of both worlds. Superalloys have better bulk mechanical properties, but oxidation and corrosion only happen at the surface. With a TBC covering the surface, the superalloy has the oxidation resistance of a ceramic!
Oxidation resistance is a large limiting factor for superalloys, but ceramics also have a high melting point and low conductivity. This means that the TBC can insulate the superalloy from high temperatures.
Suppose that a superalloy has a maximum operating temperature of 1000°C, but a ceramic has a maximum operating temperature of 2000°C. That might mean that you could run the turbine at 1200°C, because the ceramic coating insulates the superalloy so that it only experiences 1000°C.
That’s a big difference!
How do Thermal Barrier Coatings Work?
Seems simple, right? Just slap a high-temperature ceramic onto a superally, and you have a TBC!
Well, not quite. Unfortunately, it’s more complicated than that. There are many engineering requirements for the system to work as I’ve described it.
The TBC needs to perform several different functions. It needs to protect the alloy against heat and oxidation. Furthermore, it needs to “stick” to the alloy (not trivial!) and have a similar coefficient of thermal expansion, so the coating doesn’t fall off when the turbine is heated or cooled.
Finally, the TBC needs to avoid diffusing with the superalloy. Diffusion happens relatively fast at these high temperatures, and diffusion could change the compositions and mess up properties of both the superalloy and the coating.
For the TBC to do all of this, it needs to have 3 layers. Each layer performs a different function.
The outermost layer of the TBC is the top coat. This is a porous oxide. Since it’s porous, it has very low conductivity and can survive stresses caused by changes in thermal expansion. This layer primarily insulates the alloy from high temperatures.
The innermost layer of the TBC, touching the superalloy, is the bond coat. This layer is for oxidation resistance, and serves as a barrier to diffusion. The bond coat is usually made of many metals, for example NiCoCrAlY. If these metals diffuse into the superalloy, it is not as bad as if oxygen or other detrimental elements diffuse into the superalloy. Any oxygen that gets past the top coat will react with the bond coat instead of the superalloy. In effect, the bond coat acts like a sacrificial layer which oxidizes instead of the superalloy.
The product of this reaction between the bond coat and air, called a thermally grown oxide (TGO), is the layer in between the bond coat and top coat. The TGO helps keep the other layers together, and it helps keep oxygen out. The bond coat is primarily designed to have chemistry so that an ideal TGO develops. Generally, Al2O3 is considered the best thermally grown oxide.
These 3 layers work together to keep the coating stuck to the superalloy, and to protect the superalloy from heat and oxygen.
How to Make Thermal Barrier Coatings
To make a TBC, engineers need to deposit a bond coat and top coat, the TGO will develop naturally as a result of the bond coat chemistry.
Bond Coat
Chemical Vapor Deposition (CVD) is one of the most common methods of creating a bond coat. In CVD, the substrate (base superalloy) is exposed to volatile chemical precursors, which are heated and react to form the desired deposit.
There are other methods to produce the bond coat, such as electroplating with diffusion-aluminizing, plasma spray techniques, and EB-PVD. We’ll discuss these alternatives in greater detail below when we talk about specific bond coat systems.
Top Coat
Top coats are usually produced by one of two ways: Atmospheric Plasma Spraying (APS) or Electron Beam Vapor Deposition (EB-PVD)
In APS, the coating is vaporized in plasma and shot at the substrate. The coating “splats” on the surface, forming small plates or flakes.
In EB-PVD, an ingot is blasted by electrons which send vaporized particles to the substrate. This process occurs in a vacuum and a magnetic field bends the electrons so the electron gun can stay out of the way.
It is also possible to produce top coats by SPS (Suspension Plasma Spraying), HVOF (High Velocity Oxygen Fuel), and PS-PVD (Plasma Spray Physical Vapor Deposition). These are not as common, so I won’t explain them further in this article. But that’s what the techniques are called, so you know what to search if you’re doing a research project or something.
Microstructure comparison: EB-PVD vs APS
The decision to make a top coat by EB-PVD or APS can be quite important. Below, I’ve shown the microstructure differences that depend on the coating method.
As you can see, EB-PVD typically produces column-like slats in the top coat. Within the columns, pores are very small (nano-scale).
This columnar microstructure allows the top coat to be relatively flexible and survive stresses from thermal expansion. However, these columns allow slightly increased heat transfer, and they don’t protect against oxidation.
If the top coat is deposited by EB-PVD, the bond coat must be very robust to develop a thick TGO and protect the underlying superalloy from oxidation!
APS typically produces a “flaky” (more technical term: “lamellar”) microstructure. There are many pores, cracks, and inter-splat interfaces. This irregular shape makes the coating more likely to flake off (more technical term: “spallation”).
However, since these cracks and defects don’t form a continuous path toward the superalloy, coatings produced by APS will have lower conductivity and allow less oxygen transport through the top coat.
Manufacturing Method | APS (Atmospheric Plasma Spraying) | EB-PVD (Electron Beam Physical Vapor Deposition) |
Brief Description | Top coat material is deposited on the substrate surface as “splats” (1-5μm thick, 200 – 400 μm diameter) using plasma jet | Top coat material is bombarded with electrons, then it evaporates and is deposited on the substrate surface) |
Morphology Type | Lamellar | Columnar |
Top Coat Thickness | 100 – 400μm (Typically 300 μm But Sometimes up to 600μm) | Typically 125 μm |
Thermal Conductivity | 0.8 – 1.7 W/mK | 1.5 – 2.0 W/mK |
Durability | Less durable than EB-PVD TBCs | More durable than APS-TBCs (higher mechanical compliance) |
Cost | Relatively Cheap | Expensive |
Applications | Combustors, fuel vaporizers, Stator vanes, after-burner flame holders, industrial gas-turbine engines (blades and nozzles) | Turbine blades and vanes in aircraft engines (In general, EB-PVD TBCs are used in more severe conditions where good mechanical compliance is needed) |
Pros | Lower cost Lower thermal conductivity Higher oxidation resistance | Higher thermal cycling Higher erosion resistance |
Cons | Less durable Low mechanical compliance | Higher cost Higher thermal conductivity Limits in chemical variability (vapor pressure issues) |
Depending on the deposition method, each layer can vary in thickness.
- Bond Coat (BC): approx. 100μm, usually in the 75-150μm range
- Thermally Grown Oxide (TGO): 1-10μm
- Top Coat (TC): approx. 100 – 400μm (APS) or 120μm (EB-PVD)
Materials for Thermal Barrier Coatings
Each material in a TBC needs to interact with the other layers. Let’s start with the substrate. Even though the substrate is not part of the TBC, since TBCs interact chemically with the underlying superalloy, it’s worth mentioning.
Substrate
“Substrate” means the thing which is coated. Since TBCs are a protective coating for superalloys, the substrate will be a superalloy.
Currently, Ni-based superalloys dominate the industry. There are also experimental/niche Co- or Fe-based superalloys, but Ni-based superalloys are much more mature.
Commercial superalloys usually have tons of different elements, including Ni, Co, Fe, Al, W, Hf, Mo, C, Re, Ti, and more.
The superalloy will also be air-cooled through internal hollow channels, although these channels won’t interact with the TBC.
Bond Coat: NiPtAl or MCrAlY
The bond coat is an oxidation-resistant metallic layer. Well, that is to say that it creates a TGO, which is oxidation-resistant.
There are 2 classes of bond coat alloys: Aluminides and MCrAlY.
Both of these include aluminum because we want to grow a TGO with Al2O3. Al2O3 is pretty much the most protective oxide, with high strength and very low oxygen diffusivity.
Aluminides are a combination of aluminum and another metal, usually Ni and Pt. These can be formed by chemical vapor deposition (CVD) or Electroplating with diffusion-aluminizing.
In CVD the substrate is exposed to one or more volatile precursors, which react and/or decompose on the substrate surface to produce the desired deposit
In electroplating, an electric current dissolves metal cations and deposits them on the substrate surface so they form a very thin, coherent metal coating.
In diffusion-aluminizing, the substrate and coating are heated in the presence of aluminum vapor. The aluminum diffuses from the atmosphere into the coating.
For example, a PtNiAl bond coat might be formed in 2 steps. First, Pt would be electroplated to the substrate surface. Then, the substrate and Pt coating would be heated in an aluminum-rich environment. Al diffuses from the atmosphere into the substrate surface, while Ni diffuses to the surface from deep within the Ni-rich superalloy.
As you can see, this process is partly dependent on the superalloy composition.
The other type of bond coat is MCrAlY. You’ll notice that “M” is not an element on the periodic table. When materials scientists want to refer to a system where the specific metal is not very important, we usually use the letter M (M stands for “metal”).
In the case of MCrAlY, M usually means Co, Ni, and/or Fe. For instance, NiCrAlY, NiCoCrY, or CoFeCrY would all be valid compositions of an MCrAlY bond coat. (Sometimes the Y is neglected in MCrAl coatings, but yttrium is usually very beneficial).
MCrAlY bond coats can be applied by APS or EB-PVD, just like top coats.
Thermally Grown Oxide (TGO): Al2O3
TGOs are the “secret sauce” that allow TBCs to work.
TGOs need to stick to the bond coat and the top coat. They need to act as a diffusion barrier so oxygen doesn’t get into the superalloy, and metals into the superalloy don’t get into the top coat. TGOs need to have an intermediary coefficient of thermal expansion, so that the TBC doesn’t fall off of the superalloy when it gets heated, and the TBC needs to be strong enough to withstand the stress that happens when the coefficients of thermal expansion don’t match exactly.
Luckily, TGO formation is inevitable. No matter what you do, the superalloy will interact with some oxygen and form an oxide. Unluckily, not all oxides are good (just think about rust).
The bond coat is used to control which type of TGO forms. In general, α-Al2O3 is the best TGO that has the optimal combination of properties I mentioned earlier. We want our TGO to grow slowly, uniformly, and without defects.
Top Coat: YSZ
The top coat can be made of different materials, but one material is currently way better than the others. That material is Yttria-stabilized zirconia, also called YSZ.
YSZ is a really interesting ceramic because of transformation toughening, which I’ll have to explain in another article. For the purposes of TBCs, let’s just say that YSZ is the toughest ceramic available. YSZ also has a coefficient of thermal expansion (CTE) that is close enough to superalloys’. Toughness and matching CTEs allow YSZ to survive for a long time as the top coat.
YSZ is zirconia (ZrO2) with a small amount of yttria (Y2O3) substituting for zirconia. You can read all about the effects of yttria in my upcoming article about transformation toughening, but for now, here is a cool chart showing the durability of zirconia with different amounts of yttria.
You can see that the maximum durability comes at 6-8 wt% yttria.
YSZ is also a good thermal insulator. It has a thermal conductivity of 1.2 – 1.8 W/mK. Researchers’ next goal is to go below 1 W/mK. Achieving this step is far away because it will likely require a new top coat material.
In the future, YSZ may be replaced by pyrochlore materials or perovskite-structured oxides as the best top coat. However, that future is still far away.
Final Thoughts
Thermal Barrier Coatings (TBCs) are a very important step in developing high-temperature materials. TBCs are incredibly complicated and need to conform to a ton of constraints influencing diffusivity, thermal expansion, thermal conductivity, strength, and more.
TBCs are a combination of 3 layers: bond coat, thermally grown oxide, and top coat.
The layer directly on top of the superalloy, the bond coat, is about 100μm thick. This coat can be applied with Atmospheric Plasma Spraying (APS), Chemical Vapor Deposition (CVD), or electroplating and aluminizing. The bond coat is usually a combination of metals that won’t diffuse into the superalloy, and aluminum. The aluminum is used for producing Al2O3 in the TGO.
The layer on top of the bond coat, the thermally grown oxide (TGO), is not deposited. Instead, it grows because of oxygen getting trapped in the bond coat. Al2O3 is usually the best oxide.
The outermost layer, called the top coat, is 100 – 400μm thick and usually made of YSZ. This coat can be deposited by a variety of methods such as Atmospheric Plasma Spraying (APS) or Electron Beam Physical Vapor Deposition (EB-PVD).
References and Further Reading
Peter W. Merlin Design and Development of the Blackbird: Challenges and Lessons Learned
D.R. Clarke and C.G. Levi Materials Design for the Next Generation Thermal Barrier Coatings
Nitin P. Padture, Maurice Gell, Eric H. Jordan Thermal Barrier Coatings for Gas-Turbine Engine Applications
David R. Clarke , Matthias Oechsner , and Nitin P. Padture Thermal-barrier coatings for more efficient gas-turbine engines
Sanjay Sampath , Uwe Schulz , Maria Ophelia Jarligo , and Seiji Kuroda Processing science of advanced thermal-barrier systems
Wei Pan , Simon R. Phillpot , Chunlei Wan, Aleksandr Chernatynskiy , and Zhixue Qu Low thermal conductivity oxides